1,What is a lithium battery?
A lithium battery (Lithium Cell) refers to a type of battery that uses lithium metal or lithium alloy as the negative electrode material and a non-aqueous electrolyte solution. The working principle involves the continuous insertion and extraction of lithium ions while combining with electrons. The basic structure of a lithium battery consists of five parts: positive electrode, negative electrode, separator, electrolyte, and casing. It has advantages such as high specific energy, long service life, and environmental friendliness.
Lithium batteries can be classified according to dimensions such as battery structure, number of uses, external structure, and electrode material. When classified by the number of uses, they can be divided into primary lithium batteries and secondary lithium batteries. Common performance parameters of lithium batteries include open circuit voltage, operating voltage, rated capacity, depth of discharge, self-discharge rate, battery energy, and internal resistance. Lithium batteries are widely used in various devices and fields, such as portable devices, energy storage, and industrial applications, with new energy vehicles representing one of the leading trends.
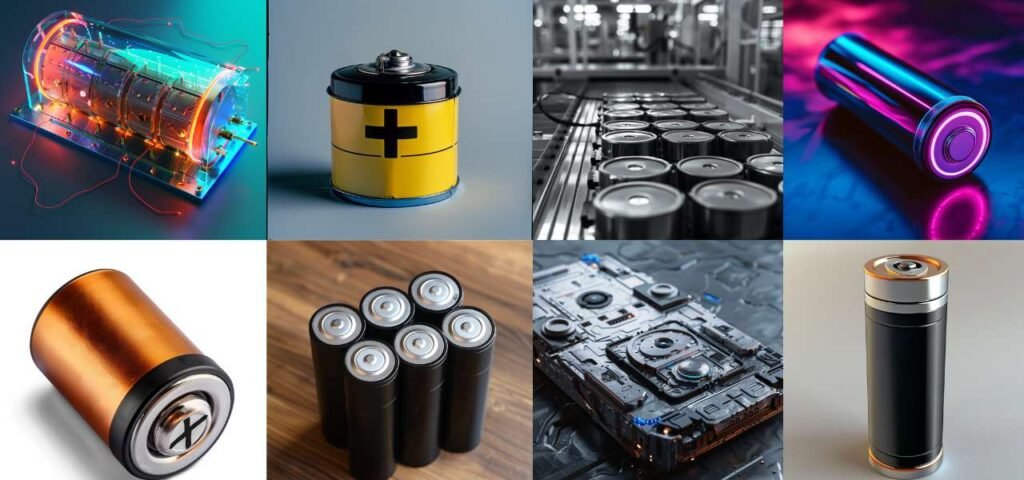
2,The History of Lithium Battery Development:
In 1913, the concept of lithium metal batteries was proposed by American chemist Gilbert N. Lewis and further researched. However, due to the highly reactive nature of lithium metal, it was unstable in air and water, which led to little attention to lithium-based electrochemical batteries for several decades until the 1960s.
The oil crisis of the 1970s drove the search for alternative energy sources, and new demands emerged in military, aerospace, medical, and other fields for power sources with higher energy density than conventional batteries could provide.
In 1958, William S. Harris proposed the use of cyclic esters as electrolytes for lithium metal batteries, providing a new direction for the study of organic non-aqueous lithium batteries.
In 1962, at a meeting of the Electrochemical Society in Boston, Chilton Jr. and Cook from Lockheed Missile and Space Co. proposed the idea of a “lithium non-aqueous electrolyte system,” which marked the birth of the prototype of lithium batteries by introducing reactive lithium metal into battery design.
Meanwhile, efforts were made to make rechargeable lithium batteries from successful experiences with primary lithium batteries. In 1965, German chemist Walter Rüdorff discovered that lithium ions could be chemically embedded in a layered structure sulfide TiS2, attracting the attention of scientists seeking reversible electrochemical lithium storage, such as Stanley Whittingham.
In the early 1970s, the United States NASA and Japan’s Matsushita Electric Industrial Co. developed a primary battery using fluorinated graphite as the positive electrode and metallic lithium, marking the first commercialization of lithium batteries.
From 1972 to 1979, Stanley Whittingham, then a researcher at Exxon, conducted detailed studies and proved that layered metal sulfides (TiS2) could reversibly store lithium electrochemically, leading to the development of rechargeable lithium batteries from disposable ones. He built a prototype of a rechargeable lithium-metal battery based on this principle.
In 1980, French scientist Michel Armand proposed for the first time the idea of using both positive and negative electrodes with embedded lithium storage mechanisms to build a new type of secondary lithium battery system, known as the rocking chair battery, where lithium ions shuttle back and forth reversibly between the positive and negative electrodes during charging and discharging processes. However, the term “lithium-ion battery” did not exist at that time.
In 1980, John B. Goodenough, then a professor at the University of Oxford’s Inorganic Chemistry Department, proposed using lithium-containing metal oxides as positive electrodes instead of lithium-free metal sulfides, which had higher voltage and chemical stability. After extensive research and exploration, he eventually found layered lithium cobalt oxide (LiCoO2, discharge voltage: 3.7 V, stable in air), which provided an ideal positive electrode material for building the prototype of a rocking chair lithium-ion battery.
In 1982, Dr. Yazami demonstrated for the first time that graphite could reversibly store lithium electrochemically in polymer electrolytes without liquid organic solvents, confirming the feasibility of using graphite carbon as the negative electrode material for lithium-ion batteries. Subsequently, in 1983, Professor Akira Yoshino of Asahi Kasei Corporation proposed a prototype of a lithium-ion battery using lithium cobalt oxide as the positive electrode and polyacetylene as the negative electrode. However, due to the low density and capacity of polyacetylene and its poor chemical stability, Professor Akira Yoshino began to search for more carbon-based materials. In this exploration process, he discovered certain carbon materials with special crystal structures (carbon nanowires grown by vapor-phase deposition) that could avoid co-intercalation and had higher capacity. Following this research direction, he eventually found petroleum coke as the negative electrode and used it with lithium cobalt oxide as the positive electrode to construct the world’s first lithium-ion battery model.
In 1983, M. Thackeray, J. Goodenough, and others discovered that spinel manganese oxide is an excellent positive electrode material with low cost, stability, and excellent conductivity and lithium-ion conductivity. Its decomposition temperature is high, and its oxidative capacity is much lower than that of lithium cobalt oxide, thus avoiding the danger of combustion and explosion even in the event of short circuits or overcharging.
In 1988, Canada’s Moli Energy Corporation launched the first commercial lithium-ion battery (Li/MoS2), which attracted widespread attention from the industry. However, despite the successful demonstration of reversible lithium-ion chemistry in principle, the tendency of lithium metal negative electrodes to form dendritic lithium deposits during repeated cycling leading to internal short circuits and fires or explosions was a major concern.
In 1989, due to fire and explosion incidents, Moli Energy Corporation had to recall its battery products on a large scale. Subsequently, other battery giants such as Sony, Sanyo, and Panasonic also decided to terminate their research and development of secondary lithium metal batteries, bringing an abrupt end to the commercialization of secondary lithium metal batteries.
In the following years, Professor Akira Yoshino, working with scientists from Sony Corporation, focused on developing commercial lithium-ion batteries. In June 1991, Sony Corporation introduced the first commercial lithium-ion battery (positive electrode: lithium cobalt oxide, negative electrode: petroleum coke, electrolyte: LiPF6-PC), marking the beginning of the era of lithium-ion batteries.
In 1996, Padhi and Goodenough discovered phosphates with olivine structure, such as lithium iron phosphate (LiFePO4), which are safer than traditional positive electrode materials for lithium-ion batteries, especially resistant to high temperatures and overcharging, far exceeding the performance of traditional lithium-ion battery materials. Therefore, they have become the mainstream positive electrode materials for high-current discharge power lithium-ion batteries.
In the 1990s, lithium-ion batteries for personal devices such as mobile phones and laptops were launched. Initially used by the mobile phone industry, they were later widely used in portable speakers, laptops, and other devices.
In 1998, the Tianjin Institute of Power Sources in China began commercial production of lithium-ion batteries. Conventionally, lithium-ion batteries are also referred to as lithium batteries, but these two types of batteries are different. Lithium-ion batteries have become mainstream.
After 2006, the ET (Environment & Energy) revolution drove the surge in demand for electric vehicles, and lithium-ion batteries with performance suitable for automotive secondary batteries, such as high voltage and large energy density, were also used for electric vehicle-related purposes. In the following days, lithium-ion batteries continued to advance and were widely used in various commodities, flourishing to this day.
In October 2018, Professor Liang Jiajie and Professor Chen Yongsheng’s team from Nankai University in China collaborated with Professor Lai Chao’s team from Jiangsu Normal University to successfully prepare a multi-level structured silver nanowire-graphene three-dimensional porous carrier, which loaded metallic lithium as a composite negative electrode material. This carrier can suppress the growth of lithium dendrites, thereby achieving ultra-fast charging of batteries and greatly extending the “life” of lithium batteries.
In 2020, 80-year-old Chen Liqian, China’s first lithium battery expert, and his team developed a new type of battery material—nano-silicon lithium batteries, which have a capacity five times that of traditional lithium batteries.
In November 2021, the team led by Dr. Wang Zhaoyang, a member of the National Academy of Inventors in the United States, invented an all-weather battery that can solve the problem of lithium batteries being intolerant to low temperatures. This technology was adopted for the 2022 Beijing Winter Olympics and became one of the core technologies driving electric vehicles for the Olympics.
In 2023, Professor Su Xin’s team from the Advanced Lithium Battery Technology Research Center at Harbin Institute of Technology (Weihai), China, developed a new technology that not only increases the lifespan of lithium batteries by 20% but also maintains a battery capacity decrease of less than 20% in extremely low-temperature environments of minus 43 degrees Celsius (°C).
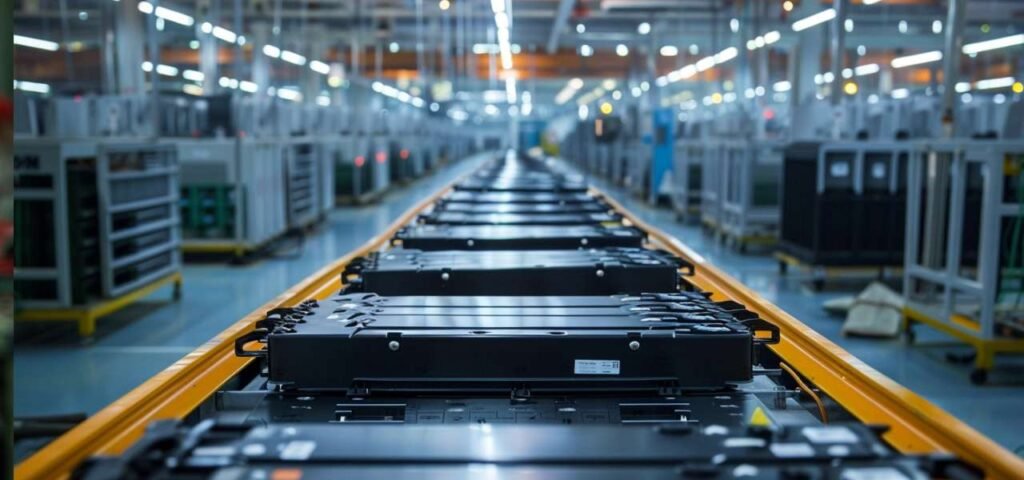
3,Basic Structure of Lithium Battery
Positive Electrode
The types of positive electrode materials can be roughly divided into lithium cobaltate (LiCoO2), lithium manganate (LiMn2O4), lithium iron phosphate (LiFePO4), etc. The selection of positive electrode materials directly affects the performance of lithium batteries during operation. During charging and discharging, lithium ions at the positive electrode undergo insertion and extraction reactions.
Negative Electrode
Negative electrode materials are roughly divided into two types: tin-based and alloy-based negative electrode materials, which are still in the experimental stage and have not been widely used in commercial products. The second type is carbon-based negative electrode materials, usually graphite, which is widely used in practice. It is a safe, non-toxic material with a long cycle life. It has a high specific capacity and reversibility during charging and discharging, thereby maintaining good stability during battery operation.
Separator
The main materials of the separator are polyethylene (PE) obtained by wet process and polypropylene (PP) obtained by dry process. It has the functions of permeability and protection, placed between the positive and negative electrodes. The pore size of the separator should ensure that lithium ions can pass normally during battery operation, while preventing the penetration of other substances, effectively avoiding the occurrence of internal short circuits caused by direct contact between the two electrode active materials. It can maintain sufficient stability under external forces such as puncture and stretching.
Electrolyte
The electrolyte is mainly composed of lithium salt, organic solvent, and necessary additives. It ensures better transmission of ions between the positive and negative electrodes, maintaining the strength of the battery during continuous operation. In high or low-temperature conditions, the electrolyte of lithium batteries basically determines the cycling life and safety performance of the battery.
Casing
The casing protects the internal materials of the battery. It is lightweight and has a series of high-strength characteristics such as explosion-proof, high temperature resistance, and corrosion resistance, effectively suppressing the polarization reaction of the battery, enhancing the consistency of lithium batteries, and thus improving the cycle life.
4,Key Materials of Lithium Battery
Positive Electrode Material
Positive electrode materials account for 20%-30% of the production cost of lithium batteries and are an important factor determining the performance of lithium batteries. The main technical routes include lithium cobaltate, lithium manganate, lithium iron phosphate, ternary materials, etc. With the advancement of technology, high-nickel ternary materials with energy density advantages and lithium manganese iron phosphate with safety and economic advantages are the main development directions.
Negative Electrode Material
As the carrier of lithium ions and electrons, negative electrode materials mainly bear the functions of energy storage and release, which directly affect the energy density, cycle life, safety, and fast charging capability of batteries. Currently, artificial graphite is the main negative electrode material, accounting for about 10%-15% of the cost of lithium-ion batteries.
Electrolyte
The electrolyte is composed of solvent, lithium salt, and electrolyte. The most important components are lithium salt and additives, accounting for about 12% and 8%, respectively. The electrolyte has a significant impact on the comprehensive performance of lithium batteries, such as high and low-temperature performance, rate performance, and safety performance.
Separator
The lithium battery separator is an important material that determines the performance, safety, and cost of the battery. Among the four major lithium-ion battery materials, the cost of the separator accounts for only second to the positive electrode material, about 10%-15%, and even higher in some high-end batteries. The separator is located between the positive and negative electrodes of the lithium battery. Its main function is to separate the positive and negative electrode active materials to prevent short circuits caused by contact between the two electrodes. Additionally, during electrochemical reactions, the separator can also maintain the necessary electrolyte and form a channel for ion movement.
5,Technical Parameters of Lithium Battery
Open Circuit Voltage: Open circuit voltage (OCV) refers to the potential difference between the positive and negative electrodes of the battery when no current is passing through. The open circuit voltage is related to factors such as the activity of the positive and negative electrode materials, electrolyte solution, and environment, rather than the size and internal structure of the battery. In the battery management system, open circuit voltage can also predict the state of charge (SOC) of the battery, which is measured through constant current charge and discharge experiments.
Operating Voltage: Operating voltage, also known as battery terminal voltage, refers to the potential difference between the positive and negative electrodes of the battery when a load is connected. During the discharge process of the battery, the terminal voltage is lower than the open circuit voltage, while during the charging process, it is higher than the open circuit voltage. The terminal voltage of the battery is also affected by temperature and discharge rate. Generally, the higher the temperature, the faster the terminal voltage rises; the higher the discharge rate, the more the terminal voltage drops.
Rated Capacity: The rated capacity refers to the minimum amount of electricity that the battery can discharge under the designed and manufactured conditions.
Depth of Discharge (DOD): The depth of discharge refers to the extent of discharge during battery discharge, also expressed as the percentage of rated capacity discharged within a certain period. DOD usually appears in combination with state of charge (SOC), with the two being interrelated. SOC is the ratio of the remaining capacity of the battery to the fully charged capacity. When the battery discharges the same amount of charge as the rated capacity, the SOC will be reduced to 0, and the DOD of the battery will be 100%.
Discharge Rate: The discharge rate refers to the current value required for the battery to discharge the nominal capacity within the specified time. In the same time period, the discharge amount is generally an integer or fraction of the rated capacity of the battery, and it is also an important parameter to characterize the power characteristics of the battery. For example, discharging a battery with a capacity of 30Ah at a discharge rate of 1C means discharging the battery with a current of 30 amperes.
Self-discharge Rate: The self-discharge rate, also known as charge retention capacity, refers to the ability of the battery to maintain its charge in a stable state. The self-discharge rate of lithium batteries is related to factors such as the purity of raw materials during processing, manufacturing processes, and environmental temperature. At room temperature, the self-discharge rate of lithium batteries is 5%-8%. When the temperature is higher, for a given self-discharge current, the SOC value of the battery will be lower. When the self-discharge current is higher, for a given temperature, the SOC value of the battery will be higher, and the battery will consume more electricity when both the temperature and the self-discharge current are high.
Battery Energy: The battery energy refers to the ability of the battery to convert chemical energy into electrical energy under certain discharge conditions. Its unit is usually expressed in watt-hours (Wh) or kilowatt-hours (kWh).
Internal Resistance of the Battery: The internal resistance of the battery refers to the resistance encountered when the current passes through the battery during charging and discharging processes. The resistance is not a fixed value; it changes continuously during the charging and discharging process and is an important indicator of battery performance. The resistance is one of the important indicators to describe the health status of the battery. During charging and discharging, the internal resistance of the battery affects the terminal voltage and open circuit voltage of the lithium battery, and the internal resistance also changes continuously, affected by factors such as electrolyte concentration, environmental temperature, and electrochemical reactions. It is generally divided into ohmic resistance and polarization resistance. Ohmic resistance exists in the electrodes and electrolyte of the battery, which will cause the lithium-ion battery to be unable to fully equivalent to an ideal power source. The resistance generated by the internal movement of the battery is called ohmic resistance. Ohmic resistance is one of the important parameters of lithium batteries, and its size is related to factors such as battery type, battery structure, and electrolyte concentration. During the charging and discharging process of lithium batteries, the positive and negative electrodes of the battery undergo chemical reactions to generate polarization resistance.

6,Working Principle of Lithium Battery
The working principle of a lithium battery is the continuous insertion and extraction reactions of lithium ions, combined with electrons. Lithium is the most easily ionized, and the electrolyte between graphite and metal oxides acts as a protection. During charging, the separator only allows Li+ to pass. The positive side of the power source attracts and repels electrons and ions from lithium atoms in metal oxides, and e – cannot pass through the electrolyte, flowing through the external circuit to reach the graphite layer, Li+ is attracted to the negative electrode due to its own characteristics and passes through the separator to reach the graphite space of the negative electrode. When all lithium atoms reach the graphite layer, it means the battery is fully charged, Li+ and e – are detached from the positive electrode oxide structurally. During discharge, Li+ wants to be restored to a stable state as part of the positive electrode oxide. Due to this tendency, Li+ passes through the electrolyte and moves back to the positive electrode, and e – also moves in the opposite direction to maintain the charge balance of the positive electrode, thereby supplying power externally.
7,Charging Methods of Lithium Battery
There are two main charging methods for lithium batteries, mainly constant current charging mode and constant voltage charging mode. Whether it is constant current charging mode or constant voltage charging mode, the charging process can mainly be divided into four stages: trickle charging (low-voltage pre-charging), constant current charging, constant voltage charging, and charging termination.
First stage: Trickle charging. It mainly applies trickle charging (restorative charging) to battery cells that are completely discharged, that is, trickle charging is used when the battery voltage is below 3V. The trickle charging current is one-tenth of the current under constant current charging mode, that is, 0.1C (C is a representation method of current with respect to the nominal capacity of the battery, for example, if the capacity of the battery is 1000 mA·h, then 1C represents a charging current of 1000mA).
Second stage: Constant current charging. When the battery voltage rises above the threshold of trickle charging, the charging current is increased to perform constant current charging. Generally, the current of constant current charging is in the range of 0.21.0C. At this time, the battery voltage will gradually increase with the constant current charging process. Generally, the voltage set for a single cell battery is 3.04.2V.
Third stage: Constant voltage charging. When the battery voltage reaches 4.2V, the constant current charging stage ends, and the constant voltage charging stage begins. At this time, the change in current depends on the saturation degree of the battery core. As the charging process continues, the charging current gradually decreases from the maximum value. When it decreases to 0.05C, it is considered that the charging is terminated.
Fourth stage: Charging termination. There are two main methods for terminating charging. The first method uses the minimum charging current to determine or use a timer (or a combination of both). The minimum current method is used to monitor the charging current during the constant voltage charging stage, and the charging is terminated when the charging current decreases to 0.05C (or a value in the range of 0.02~0.07C). The second method uses a timing method, with the starting time of the constant voltage charging stage as the initial time, and the charging process is terminated after continuous charging for 2 hours.
Completely discharged batteries require 2.5~3 hours to complete the above four stages of charging. After charging is complete, if the battery voltage is detected to be below 3.89V, it will be recharged.
8,Main Classifications of Lithium Batteries
According to Battery Structure
Lithium batteries can be broadly categorized into two types based on their structure: lithium metal batteries and lithium-ion batteries.
The main difference between lithium metal batteries and lithium-ion batteries lies in the negative electrode. In lithium metal batteries, the negative electrode is metallic lithium, while in lithium-ion batteries, materials such as graphite, silicon, and lithium titanium oxide (commonly known as “host” materials) are used as the negative electrode. Lithium metal batteries include primary batteries and secondary batteries, such as coin cells and button cells. Lithium metal batteries are commonly used in applications such as watches, calculators, and cameras.
Lithium-ion batteries mainly consist of a series of batteries with positive electrodes made of ternary materials and negative electrodes made of materials such as graphite, silicon, and lithium titanium oxide. Due to the safety issues associated with the deposition of lithium dendrites during the charging process of lithium metal batteries, which can cause short circuits and safety problems, lithium-ion batteries are currently more widely used. Lithium-ion batteries are commonly used in the consumer electronics industry, such as mobile phones and laptops.
According to Usage Frequency
Lithium batteries are divided into primary batteries (non-rechargeable) and secondary batteries (rechargeable), which can be used multiple times.
Primary batteries, also known as disposable batteries, can only convert chemical energy into electrical energy once and cannot be restored to chemical energy (or have very poor reversibility). Non-rechargeable lithium batteries come in various types, with commonly used ones including lithium-manganese dioxide batteries, lithium-thionyl chloride batteries, and lithium batteries with other compounds.
Secondary batteries, also known as rechargeable batteries, can convert electrical energy into chemical energy for storage and then convert it back into electrical energy when needed. Rechargeable lithium batteries come in various types, such as lithium-vanadium oxide batteries, lithium-ion batteries, and newly developed lithium-polymer batteries abroad. Lithium-ion batteries are the most widely used batteries in mobile phones currently, but they should not be overcharged or overdischarged during use, as it can damage the battery or render it unusable. Therefore, lithium-ion batteries are equipped with protection components or circuits to prevent expensive battery damage. The charging requirements for lithium-ion batteries are high, with the need to ensure that the termination voltage accuracy is within 1%. Major semiconductor device manufacturers have developed various lithium-ion battery charging ICs to ensure safe, reliable, and fast charging.
According to External Structure
Based on the external structure, batteries can be classified into three types: pouch lithium batteries, cylindrical lithium batteries, and prismatic lithium batteries.
According to Electrode Material
Lithium Iron Phosphate (LFP) Battery: The lithium iron phosphate battery uses lithium iron phosphate as the positive electrode material. Also known as lithium iron phosphate lithium-ion battery, it is particularly suitable for power applications due to its performance, hence also referred to as lithium iron phosphate power battery or lithium iron power battery. In the metal trading market, cobalt (Co) is the most expensive, but with limited reserves, while nickel (Ni) and manganese (Mn) are relatively cheaper, and iron (Fe) is the cheapest. The price of positive electrode materials also follows the price trends of these metals. Therefore, batteries made with positive electrode materials should be the cheapest.
Lithium Cobalt Oxide (LCO) Battery: The lithium cobalt oxide battery has stable structure, high specific capacity, and outstanding comprehensive performance. However, it has poor safety and high cost. It is mainly used in small and medium-sized battery cells and is widely used in small electronic devices such as laptops, mobile phones, MP3/MP4 players, etc. with a nominal voltage of 3.7V.
Lithium Manganese Oxide (LMO) Battery: The lithium manganese oxide battery refers to batteries with lithium manganese oxide as the positive electrode material. The nominal voltage of lithium manganese oxide batteries ranges from 2.5V to 4.2V. Lithium manganese oxide is a low-cost, safe, and has good low-temperature performance positive electrode material, hence it is widely used. However, its material itself is not very stable and tends to decompose to produce gas. Therefore, it is often mixed with other materials to reduce the cost of battery cells, resulting in faster cycle life decay, swelling, poor high-temperature performance, and relatively short lifespan. It is mainly used in large and medium-sized battery cells for power batteries, with a nominal voltage of 3.7V.
Ternary Polymer Battery: The ternary polymer lithium battery refers to lithium batteries with ternary materials such as nickel cobalt manganese lithium or nickel cobalt aluminum lithium as the positive electrode material. The nominal voltage of this battery has reached 3.7V, and its capacity has reached or even exceeded that of lithium cobalt oxide batteries.
Lithium Titanium Oxide (LTO) Battery: The lithium titanium oxide battery is a lithium-ion secondary battery with lithium titanium oxide as the negative electrode material, which can be used in combination with positive electrode materials such as manganese oxide, ternary materials, or lithium iron phosphate to form lithium-ion secondary batteries with a voltage of 2.4V or 1.9V. Additionally, it can also be used as the positive electrode to form lithium-ion secondary batteries with a voltage of 1.5V when combined with metallic lithium or lithium alloys.
9,Key Characteristics of Lithium Batteries
Advantages
High energy density: Energy density refers to the energy per unit mass or volume, measured in Wh/kg or Wh/L. Lithium batteries have a high storage energy density, currently reaching 460600 Wh/kg, which is 67 times that of lead-acid batteries.
Long service life: The service life can exceed 6 years. Using lithium iron phosphate as the positive electrode material, batteries discharged and recharged at 1C (100% depth of discharge, 100% DOD) have a record of being usable for up to 10,000 cycles.
High rated voltage: The working voltage per cell is 3.7V or 32V, which is approximately equal to the series voltage of 3 or more nickel rechargeable batteries, facilitating the formation of battery power packs.
High power handling capability: Lithium iron phosphate batteries used in electric vehicles can achieve a charging and discharging capability of 15~30C, facilitating high-intensity starting and acceleration.
Low self-discharge rate: This is one of the most outstanding advantages of these batteries. Lithium batteries charge quickly, reaching optimal status in just 1~2 hours. Additionally, leakage is rare, and the self-discharge rate is generally below 1% per month, less than 1/20 of nickel-metal hydride batteries.
Lightweight: The weight per unit volume is approximately 1/6~1/5 of lead-acid batteries.
Strong temperature adaptability: They can be used in environments ranging from -20 to 60 degrees Celsius, and with proper processing, can even be used in environments as cold as -45 degrees Celsius.
Environmentally friendly: They do not contain or produce toxic heavy metal elements and substances such as lead and mercury during production, use, or disposal. The production process consumes minimal water, contributing significantly to water resource conservation.
No memory effect: Lithium batteries can be partially charged and discharged without reducing their capacity.
Disadvantages
High internal impedance: Due to the organic solvent electrolyte used in lithium batteries, their conductivity is much lower than that of nickel-cadmium and nickel-metal hydride battery electrolytes, resulting in an internal impedance about 11 times higher than that of nickel-metal hydride batteries and nickel-cadmium batteries.
Large voltage variation during operation: When discharged to 80% of rated capacity, the voltage variation of nickel batteries is minimal (about 20%), while lithium batteries have a larger voltage variation (about 40%), which is a serious drawback for battery power supply. However, due to the high discharge voltage of lithium batteries, the remaining capacity of the battery can be easily detected.
High cost: The electrode material is relatively expensive.
Strict assembly requirements for lithium batteries: Low humidity conditions are required for assembly, and the battery structure is complex, requiring special protective circuits to prevent overcharging or over-discharging of the battery.
Poor safety: The use of organic electrolytes in lithium batteries poses certain safety hazards.
10,Current Situation and Transportation Safety of Lithium Batteries
Current Situation
Lithium batteries have high energy density and are prone to fire and explosion accidents. Since their commercial introduction, many incidents have occurred: in November 2005, Nikon urgently recalled a digital camera lithium-ion battery due to issues like battery explosion, overheating, and melting. In August 2006, computer manufacturers Dell and Apple announced the recall of millions of laptop lithium-ion batteries due to safety concerns. In 2016, Samsung’s flagship smartphone Galaxy Note 7 experienced over thirty explosions and fires caused by battery defects within a month of its launch, leading to the discontinuation of the product. On May 7, 2017, a truck carrying 18650 lithium-ion batteries caught fire in Shenzhen’s Huacheng logistics center, suspected to be caused by battery collision during transportation, and so on.
Transportation Safety
The danger of lithium batteries mainly depends on the lithium content, as lithium is a reactive metal that is flammable and reacts violently with water, releasing hydrogen gas. Improper handling during transportation can lead to battery damage, resulting in high temperatures, and even combustion and explosion. Therefore, the transportation safety of lithium batteries is increasingly emphasized.
In 1956, the “Recommendation on the transport of dangerous goods (TDG)” was first published by the United Nations Economic and Social Council’s Committee of Experts on the Transport of Dangerous Goods, providing authoritative regulations on battery transportation safety, and serving as the fundamental requirement for battery transportation safety. This committee was reorganized in 2001 and renamed as the “Committee of Experts on the Transport of Dangerous Goods and on the Globally Harmonized System of Classification and Labelling of Chemicals.”
In 2017, the International Air Transport Association (IATA) released the Dangerous Goods Regulations (DGR) 58th edition (2017), which includes specific requirements for the transportation of lithium batteries.
In January 2017, China began implementing the “International Maritime Dangerous Goods Code” (IMDG) 37-14 edition issued by the International Maritime Organization, which includes content related to lithium batteries.
In 2023, the China National Railway Administration, the Ministry of Industry and Information Technology, and the China State Railway Group Co., Ltd. issued the “Guidance on the Railway Transportation of Consumer Lithium Battery Goods,” which explicitly states that qualified consumer lithium battery goods are not considered dangerous goods during railway transportation.
11,Key Technologies of Lithium Batteries
The production process of lithium batteries is divided into three main stages, including electrode preparation, cell production, and battery assembly. In the production process of lithium batteries, electrode preparation is the foundation of all processes and the beginning stage of lithium battery project technology. The production of lithium battery cells is the core of the entire process and the most critical part, while the last step is battery assembly, which has a significant impact on the quality of the finished lithium battery product. Specifically, the production process of lithium batteries includes positive electrode slurry coating, negative electrode slurry coating, positive electrode sheet production, negative electrode sheet production, shell assembly, cell filling, testing, and packaging.
Humidity Control: The humidity load parameters of lithium battery process equipment need to be maintained within a certain range, requiring the production environment to be dry air, and the sealing performance of air supply ducts and the enclosure of production workshops are also crucial influencing factors.
Thermal Pressing of Lithium Batteries: The battery separator, as the core component of the battery, plays a crucial role in isolating the conduction of positive and negative electrodes and allowing the reciprocating passage of lithium ions between the electrodes. The microporous structure on the separator is the important channel for these ions to shuttle between the positive and negative electrodes. Its gas permeability directly affects the battery’s performance. (Gas permeability of the separator refers to the amount of gas passing through the separator under a certain pressure in a certain time). If the gas permeability of the separator is poor, it will affect the transfer of lithium ions between the positive and negative electrodes, thereby affecting the charge and discharge of the lithium battery. The testing process involves fixing the battery separator, applying pressure on one side of the separator, measuring the pressure drop and the time used, and testing the air permeability of the separator. The shorter the time used, the better the air permeability.
Conductive Coating: Also known as pre-coating, in the lithium battery industry, it usually refers to a layer of conductive coating applied to the surface of the positive electrode current collector – aluminum foil. Aluminum foil coated with conductive coating is called pre-coated aluminum foil or simply coated aluminum foil. Its earliest experiments in batteries can be traced back to the 1970s, and with the development of the new energy industry, especially the rise of lithium iron phosphate batteries, it has become a popular new technology or new material.

12,Recycling and Policies of Lithium Batteries
The handling of failed lithium-ion batteries mainly includes chemical methods and mechanical methods (physical methods):
In the chemical treatment method, the typical process includes crushing, electrolyte treatment, roasting, magnetic separation, fine grinding, classification and screening, and then smelting to produce high-grade cobalt alloy, followed by wet processing to produce metallic cobalt or cobalt carbonate and lithium carbonate. The typical process of mechanical method (physical method) includes crushing, electrolyte treatment, heat treatment, magnetic separation, fine grinding, followed by classification, screening, and separation to produce copper-containing waste and refined cobalt.
China attaches great importance to the recycling of new energy vehicle power batteries and has introduced a series of regulations and standards for battery recycling. These standards and specifications include the “Interim Measures for the Management of Recycling and Utilization of Waste Power Batteries for New Energy Vehicles,” the “Industry Standard Conditions for Comprehensive Utilization of Waste Power Batteries for New Energy Vehicles,” the “Specification for the Storage and Transportation of Battery Scrap” (GB/T 26493-2011), the “Requirements for Recycling and Disposal of Communication Ion Batteries” (GB/T 22425-2008), the “Code for Management of Waste Batteries” (WB/T 1061-2016), the “Detection of Residual Energy for Automotive Power Battery Recycling” (GB/T 34015-2017), and the “Dismantling Specification for Automotive Power Battery Recycling” (GB/T 33598-2017), etc.
Among them, the “Industry Standard Conditions for Comprehensive Utilization of Waste Power Batteries for New Energy Vehicles” issued in 2019 makes clear provisions on the comprehensive utilization of waste power batteries, requiring enterprises engaged in recycling to carry out research and application of recycling technology, equipment, and processes in terms of positive and negative electrode materials, separators, electrolytes, etc., to improve the level of waste power battery recycling. Major valuable metals such as nickel, cobalt, and manganese should have a comprehensive recovery rate of not less than 98%, and the recovery rate of lithium should not be less than 85%. The comprehensive recovery rate of other major valuable metals such as rare earths should not be less than 97%. If the material repair process is adopted, the material recovery rate should not be less than 90%. The recycling rate of process wastewater should be above 90%.
13,Application Areas of Lithium Batteries
Transportation Sector
Hybrid electric vehicles mainly use lead-acid and nickel-hydrogen batteries as the main power source, with dozens of electrochemical capacitors connected in parallel as standby power sources. With the increase in automotive electronic control circuits, there is a demand for standby power sources with higher capacity. Compared with current electrochemical capacitors, new lithium-ion batteries also have high reliability and can significantly reduce space and weight, gradually replacing traditional lead-acid and nickel-hydrogen batteries. Additionally, as automobile pollution becomes more severe, measures to control and mitigate emissions, noise, etc., have become necessary, especially in densely populated and traffic-congested cities. Lithium batteries, due to their pollution-free, low-pollution, and energy-diversified characteristics, have received significant development in the electric vehicle industry, which can improve environmental pollution conditions.
Electronics Sector
Due to the high volumetric energy density of lithium batteries, they can be made smaller and lighter, thus widely used in portable electronic products. With the popularity of mobile phones, digital cameras, camcorders, laptops, and handheld game consoles, lithium battery products have maintained rapid growth and occupied the majority of the market share. With the improvement of high-current charging and discharging performance, lithium batteries will also expand their applications in wireless telephones and power tools.
Aerospace Sector
Due to the strong advantages of lithium-ion batteries, they can provide support for correction during launch and flight in the aerospace sector, as well as ground operations, and contribute to improving the efficiency of primary batteries and supporting nighttime operations.
Military Sector
Due to the high energy density, lightweight, and small size of lithium-ion batteries, they can improve the flexibility of weapons and equipment after assembly. Therefore, in the field of national defense and military, lithium-ion batteries cover many arms including land (individual soldier systems, army vehicles, military communication equipment, missiles), sea (torpedoes, submarine underwater robots), air (unmanned reconnaissance aircraft), and space (satellite spacecraft).
Energy Storage Sector
Due to the high energy density and long service life of lithium batteries, they are widely used in energy storage, usually as energy storage containers for rail transit, photovoltaic power generation, smart grids, backup power supplies, and military power supplies, closely related to energy storage technology. Lithium batteries can effectively solve the intermittent nature of photovoltaic energy storage and wind energy storage, and provide strong guarantees for the endurance of electric vehicles. The application of lithium batteries in energy storage includes power generation-side energy storage applications such as solar storage power stations and wind storage power stations, user-side energy storage applications such as solar storage charging stations, household energy storage, and backup power supplies, and energy storage applications at substations, mainly reflected in substation energy storage and virtual power plants.
14,The Development Trends of Lithium Batteries
Application Level
The lithium battery industry is an important direction for the global development of high-tech industries. Lithium batteries have excellent electrochemical stability, high energy density, high specific power, high conversion efficiency, long service life, pollution-free, and maintenance-free advantages, making them the development direction of future power batteries. Most areas of the automotive industry regard lithium batteries as the main power source for vehicles, and when combined with vehicle power, they can be transformed into electric vehicles or hybrid electric vehicles. This will lead to more and more new energy vehicles entering the lives of the public. This not only meets the requirements of green travel and low-carbon life but also reduces atmospheric pollution caused by vehicle exhaust emissions, further promoting the progress and development of related green automotive industries. In addition, through innovation and the development of advanced lithium battery technology, lithium batteries can be applied to more related technological fields, such as military or aerospace flights. Similarly, lithium batteries can also be useful as satellite batteries for small satellites, enabling these small satellites to fulfill their functions, reduce the occurrence of faults, and ensure the lithium battery as the satellite battery for a long period in the future.
Technological Level
With the improvement of China’s scientific and technological research and development capabilities, emerging technologies of lithium batteries such as solid-state lithium batteries, lithium-sulfur batteries, etc., are in a rapid development stage. In the future, the maturity and application of these technologies will further improve the performance of lithium batteries and promote their extensive application in the field of energy storage. For example, by optimizing battery materials and manufacturing processes, the cycle life of batteries can be extended, and the maintenance costs and replacement frequency of batteries can be reduced.
References
(1),Zhou, C. (2020). Research on Composite Modification and Application of Lithium Battery Separator. Zhejiang University.
(2),Xie, K. (2013). New Generation Lithium Secondary Battery Technology. Machinery Industry Press.
(3),Wang, S., & Li, X. (2021). Equivalent Circuit Modeling and State of Charge Estimation of Lithium Batteries. Machinery Industry Press.
(4),Liang, J., Chi, H., & Zhang, H. (2020). Application and Development Trend of Lithium Batteries in Energy Storage. Power Tools.
(5),Wang, S., & Li, X. (2021). Equivalent Circuit Modeling and State of Charge Estimation of Lithium Batteries. Machinery Industry Press.
(6),Zhao, Z. (2022). Research on Health Status Prediction of Lithium Batteries for Electric Vehicles. Northeast Petroleum University.
(7),Zhao, Z. (2022). Research on Health Status Prediction of Lithium Batteries for Electric Vehicles. Northeast Petroleum University.
(8),Yang, B. (Ed.). (2022). Vehicle Electric Apparatus. Machinery Industry Press.
(9),Lan, F., & Chen, J. (2022). Key Technologies of Power Battery System for New Energy Vehicles. Machinery Industry